In the complex world of materials science and mechanical engineering, comprehending the fundamental properties of metals, such as strength and stiffness, is crucial. These properties determine how a material behaves under various load conditions and play a significant role in selecting the right material for a particular application.
This article aims to demystify the concepts of strength and stiffness, drawing distinctions between strength vs. stiffness, strength vs. hardness, and illustrating the significance of these properties in the metal industries.
Defining Strength and Stiffness
Let’s begin by clarifying the basic terms:
Strength: Strength of a material refers to its ability to withstand an applied load without failure or deformation.
Stiffness: On the other hand, stiffness of material, or in more formal terms, the modulus of elasticity, measures a material’s resistance to elastic deformation under load.
To put it simply, the stronger the material, the more load it can handle without breaking. The stiffer the material, the less it will deform under a given load.
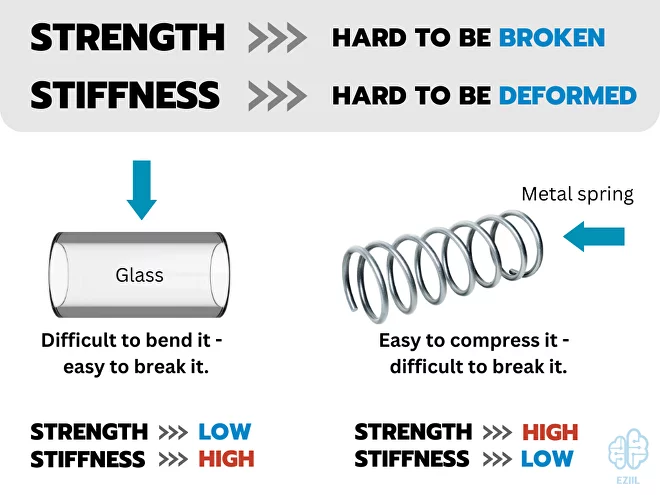
Strength vs Stiffness: The Difference
While strength and stiffness might seem similar, they serve different purposes in material science. Strength, whether it’s yield strength or ultimate strength, is about a material’s resistance to permanent deformation or fracture.
On the contrary, stiffness is about how much a material deforms under a load, but will return to its original shape once the load is removed.
Metal Strength | Metal Stiffness | |
---|---|---|
Definition | The ability of a metal to withstand an applied load without deformation or failure. It is a measure of the maximum stress a metal can endure. | The resistance of a metal to deformation under an applied load. It is a measure of how much a metal can be stretched or compressed without permanently deforming. |
Types of Strength | Yield strength, tensile strength, compressive strength, shear strength, fatigue strength, etc. | Modulus of elasticity (Young's modulus), shear modulus, bulk modulus, etc. |
Characteristics | Reflects the maximum stress a metal can handle before plastic deformation or fracture occurs. | Reflects the rigidity and resistance to deformation of a metal. |
Importance | Important in determining the structural integrity and load-bearing capacity of a metal component. | Important in designing components that require stability, minimal deflection, or precise positioning. |
Units of Measurement | Megapascals (MPa), pounds per square inch (psi), etc. | Megapascals (MPa), gigapascals (GPa), pounds per square inch (psi), etc. |
Example | A metal with high strength can withstand heavy loads without breaking or deforming. | A metal with high stiffness maintains its shape under applied forces and exhibits minimal deflection. |
Strength vs Hardness
Strength and hardness are often confused, but they are distinct properties.
While strength refers to the resistance to deformation or fracture, hardness is a measure of a material’s resistance to localised deformation, specifically, the measure of resistance to indentation or scratching.
Metal Strength | Metal Hardness | |
---|---|---|
Definition | The ability of a metal to withstand an applied load without deformation or failure. It is a measure of the maximum stress a metal can endure. | The ability of a metal to resist indentation, scratching, or wear. It is a measure of a metal's resistance to localized deformation. |
Types of Strength | Yield strength, tensile strength, compressive strength, shear strength, fatigue strength, etc. | Rockwell hardness, Brinell hardness, Vickers hardness, Mohs hardness, etc. |
Characteristics | Reflects the maximum stress a metal can handle before plastic deformation or fracture occurs. | Reflects the metal's resistance to indentation or surface deformation. |
Testing Methods | Tensile testing, compression testing, shear testing, etc. | Indentation testing (e.g., Rockwell, Brinell, Vickers), scratch testing, wear testing, etc. |
Importance | Important in determining the structural integrity and load-bearing capacity of a metal component. | Important for assessing a metal's resistance to wear, scratching, or deformation. |
Units of Measurement | Megapascals (MPa), pounds per square inch (psi), etc. | Rockwell hardness (HRC, HRB), Brinell hardness (HB), Vickers hardness (HV), etc. |
Example | A metal with high strength can withstand heavy loads without breaking or deforming. | A metal with high hardness is less prone to indentation, scratching, or wear. |
Yield Strength vs Ultimate Strength
Yield strength is the stress at which a material begins to deform plastically.
Beyond this point, the material will not return to its original shape once the load is removed. Ultimate strength, however, is the maximum stress a material can withstand before breaking.
Yield Strength | Ultimate Strength | |
---|---|---|
Definition | The stress at which a material exhibits a specified permanent deformation (typically 0.2% or 0.5% offset). It represents the onset of plastic deformation. | The maximum stress a material can withstand before fracture or complete failure occurs. |
Testing Methods | Tensile testing, where a material is subjected to an increasing load until it reaches its yield point. | Tensile testing, where a material is pulled until it fractures or breaks. |
Relationship | Yield strength is typically lower than ultimate strength, as it represents the point at which plastic deformation begins. | Ultimate strength is higher than yield strength and represents the material's maximum resistance to stress before fracture. |
Significance | Yield strength indicates the stress a material can sustain without permanent deformation. It helps determine the material's elastic behavior and deformation limits. | Ultimate strength provides an understanding of the maximum load a material can withstand before complete failure. It is crucial for designing structures to ensure they can support anticipated loads safely. |
Units of Measurement | Megapascals (MPa), pounds per square inch (psi), etc. | Megapascals (MPa), pounds per square inch (psi), etc. |
Application | Yield strength is essential for design calculations to ensure materials do not undergo excessive deformation. It is used in determining factors of safety, structural stability, and plasticity. | Ultimate strength is significant for designing components to withstand maximum expected loads without failure. It is vital in engineering applications where failure could lead to catastrophic consequences. |
Example | A material with a yield strength of 300 MPa can handle high stress levels before undergoing plastic deformation, but it may have an ultimate strength of 400 MPa before it fractures. | A material with an ultimate strength of 500 MPa can withstand significant loads before experiencing fracture, but its yield strength may be lower, such as 350 MPa, indicating the onset of plastic deformation. |
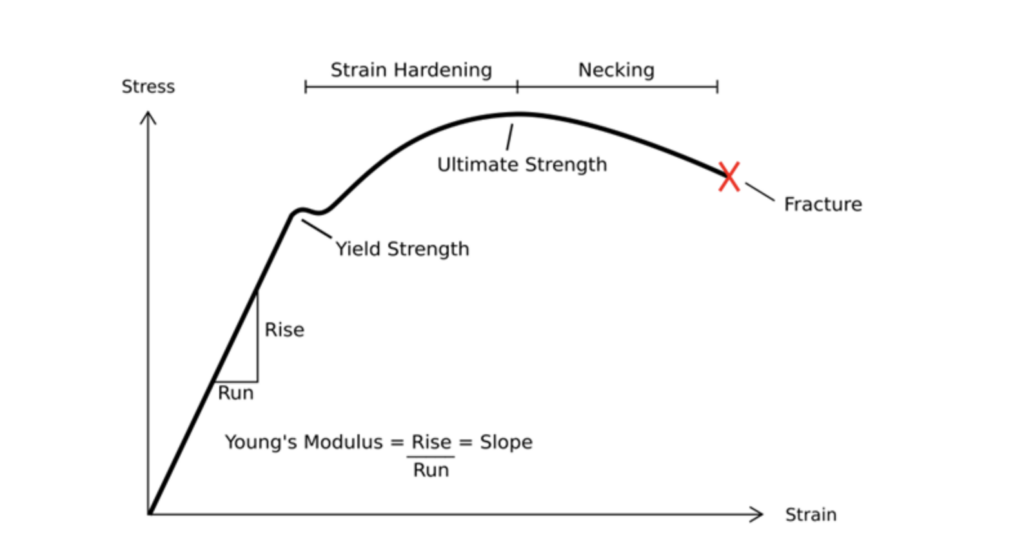
Yield Strength vs Ultimate Strength
Yield Strength vs Tensile Strength
Tensile strength, as mentioned earlier, is the resistance to breaking under tension.
It’s crucial to note that tensile strength is the highest amount of tension a material can take before breaking, which often coincides with the ultimate strength.
Yield Strength | Tensile Strength | |
---|---|---|
Definition | The stress at which a material exhibits a specified permanent deformation (typically 0.2% or 0.5% offset). It represents the onset of plastic deformation. | The maximum stress a material can withstand under tension without breaking or fracturing. |
Testing Methods | Tensile testing, where a material is subjected to an increasing load until it reaches its yield point. | Tensile testing, where a material is pulled until it fractures or breaks. |
Relationship | Yield strength is generally lower than tensile strength, as it represents the onset of plastic deformation. | Tensile strength is generally higher than yield strength and represents the maximum stress a material can endure before failure. |
Significance | Yield strength indicates the stress a material can sustain without permanent deformation. It helps determine the material's elastic behavior and deformation limits. | Tensile strength is crucial in assessing a material's ability to withstand loads without breaking or fracturing, ensuring its structural integrity and load-bearing capacity. |
Units of Measurement | Megapascals (MPa), pounds per square inch (psi), etc. | Megapascals (MPa), pounds per square inch (psi), etc. |
Application | Yield strength is important in engineering designs to ensure materials do not undergo excessive plastic deformation. It is used for calculating factors of safety, structural stability, and design limits. | Tensile strength is crucial for evaluating the suitability of materials in applications where they need to withstand tension, such as structural components, cables, or ropes. |
Example | A material with a yield strength of 300 MPa can handle high stress levels without permanent deformation, while its tensile strength may be 400 MPa, indicating the maximum stress it can endure before fracture. | A material with a tensile strength of 500 MPa can withstand significant pulling forces before breaking, while its yield strength may be lower, such as 350 MPa, indicating the onset of plastic deformation. |
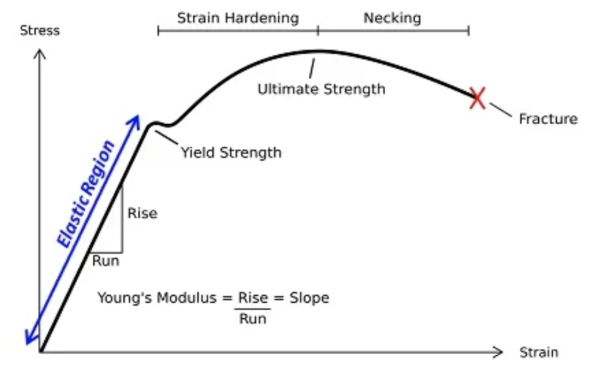
Yield Strength vs Tensile Strength
Stiffness of Steel and Ultimate Stress of Steel
Stiffness of steel: Steel, widely used in the metal industries, is renowned for its high strength and stiffness. The stiffness of steel, characterized by its modulus of elasticity, typically ranges around 200 GPa.
Ultimate stress of steel: The ultimate stress of steel, however, varies depending upon the specific grade of steel.
For instance, mild steel generally has an ultimate strength of about 370-500 MPa.
This high strength, coupled with excellent stiffness, makes steel a preferred choice for many applications.
Tensile Strength from Stress-Strain Curve
Stress-Strain curve: A stress-strain curve is a graphical representation that illustrates the relationship between stress and strain for a material. It shows how a material responds to an applied load or force and provides valuable information about its mechanical properties and behaviour.
The tensile strength can be derived from this curve as the maximum point on the stress-strain curve. This is the point at which the material will fracture or break.
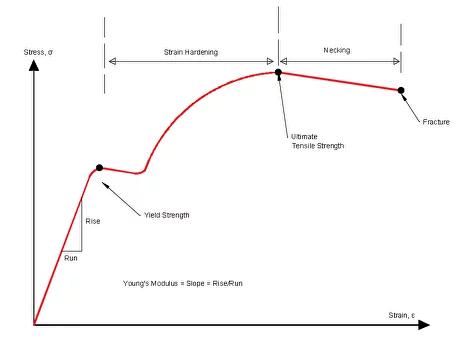
Stress strain curve
Modulus of Elasticity of Stainless Steel
The modulus of elasticity, or stiffness, of stainless steel is around 200 GPa, similar to other forms of steel.
This property makes stainless steel an excellent material for a wide range of applications, particularly where corrosion resistance, in addition to strength and stiffness, is required.
The Interplay of Strength, Stiffness, and Material Selection
In the realm of product design and mechanical engineering, understanding the interplay between strength and stiffness is critical.
The selection of materials for a specific application isn’t a one-size-fits-all approach.
It requires a comprehensive understanding of the operational demands of the component or structure, including the forces it will encounter, the nature of those forces (static or dynamic), and the acceptable amount of deformation.
For instance, a bicycle frame needs high strength to withstand the forces exerted by the cyclist and the ground, coupled with the right level of stiffness to ensure a comfortable ride.
On the other hand, a tool like a wrench requires high hardness to resist wear and tear, as well as high tensile strength to endure the forces applied during use without breaking.
Book a 60-minute demo to see
how eziil mrp solution works for you
Considering Strength and Stiffness in Metal Fabrication
When fabricating metal parts, both the strength and stiffness of the chosen metal play pivotal roles in the performance of the finished product. The fabrication process itself can also affect these properties.
Processes such as heat treatment can modify the strength and hardness of metals, enabling manufacturers to fine-tune these properties to suit specific requirements.
It’s essential to keep in mind that alterations in fabrication processes can significantly impact the properties of the end product.
The Role of Testing in Understanding Material Properties
The push towards sustainability in the metal industries has further highlighted the importance of understanding material properties. By optimising the strength and stiffness of materials, we can create products that not only perform well but also use fewer resources and have a lower environmental impact.
For example, using high-strength steel in car manufacturing can reduce the weight of the vehicle, leading to improved fuel efficiency and lower emissions.
Strength, Stiffness, and Sustainability
To ensure that a material’s strength, stiffness, and hardness are suitable for its intended application, thorough testing is essential. Techniques such as tensile testing and hardness testing provide valuable data about a material’s performance under various conditions.
These tests allow engineers to understand and predict how materials will behave in real-world applications.
Conclusion
Understanding the difference between strength and stiffness, and the interplay between these and other material properties, is critical in the field of mechanical engineering and product design, particularly in the metal industries. Whether you’re selecting materials for a project, designing a new product, or improving an existing one, a solid grasp of these concepts is essential.
Strength and stiffness are not standalone considerations but are part of a broader spectrum of material properties, including hardness, ductility, and toughness. By understanding these properties, you can make informed decisions that lead to better products, more efficient processes, and innovative solutions to engineering challenges.
In the vast and intricate world of materials science, there’s always more to learn. So, keep exploring, keep questioning, and keep pushing the boundaries of what’s possible. Whether you’re a seasoned professional or a budding engineer, your journey in this fascinating field is sure to be an exciting and rewarding one.
This article aimed to clarify the key concepts around strength and stiffness, and how they relate to each other and to other material properties. Hopefully, it has shed some light on these complex topics and will serve as a valuable resource in the future endeavours. Remember, the understanding of these basic properties is the first step towards mastery in the field of mechanical engineering and product design.